Ultra-fast photoacoustic imaging tracks hemodynamics of brain disorders in mice
Learning how the brain functions is a formidable challenge aided by the development of innovative brain-imaging technologies. Now scientists at Duke University supported with funds from the National Institute of Biomedical Imaging and Bioengineering (NIBIB) have developed an ultra-fast photoacoustic imaging system capable of visualizing functional and molecular changes in the brain related to major brain disorders.
Photoacoustic Microscopy (PAM) is a research and diagnostic tool that fires pulses of laser light into an organ of interest. The pulses cause vibrations that release an ultrasound wave, which is captured to form an image. PAM uses different wavelengths of laser light to target specific structures and even molecules in the body. For example, specific wavelengths of laser light that target hemoglobin can be used to image the array of blood vessels throughout a tissue.
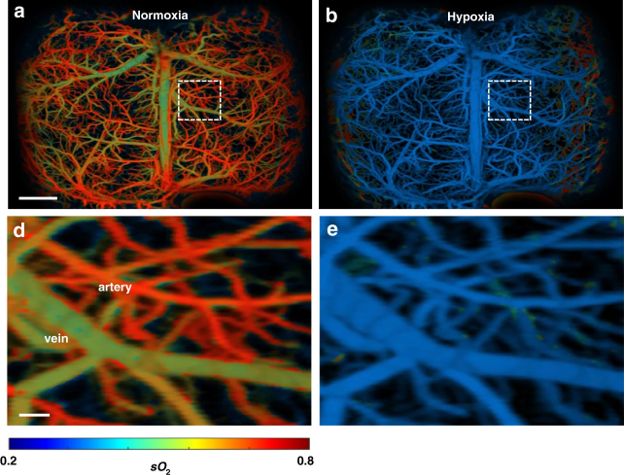
Researchers at Duke University are working to develop PAM systems that can be used to study brain functions. The challenge has been developing a PAM system that images the brain quickly enough to follow hemoglobin as it moves through the blood vessels of the brain and has a wide field of view that can track blood moving across relatively large brain regions.
The work was led by Junjie Yao, PhD, assistant professor of biomedical engineering and faculty member of the Duke Institute for Brain Sciences. Yao and his collaborators employed innovative engineering approaches to develop ultrafast functional photoacoustic microscopy (UFF-PAM), which allowed them to image functioning blood vessels across the entire mouse brain. The new system was used to study blood oxygenation, vascular constriction and dilation and other hemodynamic measures in mice in response to experimentally induced brain disorders such as stroke.
“The team at Duke and their collaborators continue to advance the promising field of photoacoustic imaging for studying brain function in disease and health,” explained Randy King, PhD, director of the program for Ultrasound: Diagnostic and Interventional at NIBIB. “This work is a significant step towards identifying vascular changes associated with important brain disorders—information that could improve treatments for diseases such as stroke, trauma and even dementia.”
A major innovation of UFF-PAM is the use of a polygon scanner—a disk with 12 flattened facets around the border. The multifaceted scanner spins at an extremely high speed above the tissue to be imaged. The spinning facets direct the pulses of laser light into the tissue and collect the returning ultrasound signals resulting in the fastest PAM imaging to date.
In a proof-of-concept experiment the researchers imaged the brain of a mouse with induced stroke. UFF-PAM images of hemoglobin in the blood are color-coded from high (shown in red-orange) to low (shown in green-blue). The stroke caused the red-orange oxygenated arteries in the brain to turn green-blue, as expected when the stroke dramatically reduced oxygen supply in the brain (hypoxia). The procedure is reversible and the return of oxygen to the brain resulted in hypoxic green-blue arteries becoming reoxygenated—returning to red-orange.
Also in the stroke model, the team, for the first time in PAM, observed a not fully understood phenomenon of great interest to researchers and clinicians. It is a wave—called a spreading depolarization (SD) wave—that emanates from the central area of stroke and moves across the brain. In the stroke model UFF-PAM detected an SD wave that caused vasoconstriction as it moved from the main stroke infarct region across the left hemisphere of the mouse brain. The wave can be seen because vasoconstriction reduces the amount of oxygenated blood in the vessels causing arteries and arterioles to go dark and then reappear as the wave progresses across the brain.
SD waves are of great interest because researchers are not fully sure of their function. “SD waves could be an indication of the level of severity of an injury, making them a potential diagnostic tool,” explained Yao. “The nature of the waves could also offer clues to the type and extent of brain injury, which could inform and optimize treatment.”
The team is now using UFF-PAM to study a number of diseases. For example, UFF-PAM was used to image the mouse placenta in response to an alcohol challenge. Surprisingly, the challenge caused a surge of oxygen into the placenta. A significant finding because the placenta functions to keep oxygen concentrations low in early gestation, which is required for proper development of the placenta and fetus. Their result suggested that fetal abnormalities may be due to a toxic increase in oxygen in response to alcohol.
While the group foresees mainly using UFF-PAM in animal models for basic research, Yao revealed plans for a handheld UFF-PAM device for use in humans. One target for such a device would be to rapidly detect sepsis—a serious blood infection. UFF-PAM could be used to monitor blood vessels beneath the skin for onset of the deadly condition, which would allow rapid, potentially life-saving treatment.
The study appears in the scientific journal Light: Science and Applications 1. The work was supported through grants R01 EB028143, R21 EB027304, R21EB027981 from the National Institute of Biomedical Imaging and Bioengineering, The National Cancer Institute, the National Institute of Neurological Disorders and Stroke, the National Heart Lung and Blood Institute, Chan Zuckerberg Initiative Grant on Deep Tissue Imaging, and by Silicon Valley Community Foundation.
-Written by Thomas Johnson, Ph.D.