Advanced microscopes yield new images of live nerve development, lend insights into brain development
Sometimes scientists discover exciting results after spending years searching for an answer to a single question. But sometimes discoveries are made by surprising collaborations and connections—resulting in answers to questions no one would have thought to ask. A paper published in Nature on February 24, 2021 has just such a story behind it.
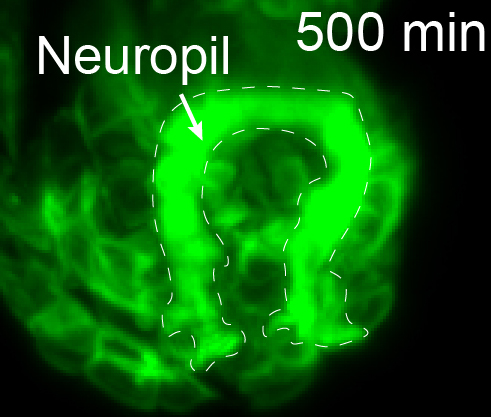
Hari Shroff and Daniel Colón-Ramos have known each other for many years and have worked on multiple scientific projects together. In some ways, it may seem like a strange partnership. Hari Shroff, Ph.D., is the chief of the Laboratory on High Resolution Optical Imaging at the National Institute of Biomedical Imaging and Bioengineering (NIBIB)—a bioengineer who invents, builds, and improves optical microscopes. With these microscopes he finds new ways to image very small objects, such as neurons and parts of cells, even when they are moving very quickly. This is not an easy task, as anyone who has taken a photo of a fast-moving object knows.
Daniel A. Colón-Ramos, Ph.D., is the McConnell Duberg Professor of Neuroscience and Cell Biology at Yale University. He studies, among other things, the brain development of a worm called Caenorhabditis elegans or C. elegans.
Shroff and Colón-Ramos began working together in 2009. As their partnership developed over the years, Shroff created some of the tools necessary to observe and track individual neurons as the C. elegans embryo develops, while Colón-Ramos studied the worm biology. The cooperation furthered both fields. Shroff made significant advances in microscopy speed and resolution as Colón-Ramos helped neurobiologists better understand how the brain forms in C. elegans, an early step that could inform how human brains develop.
In the most recent discovery to come out of their collaboration, Colón-Ramos and his team reanalyzed electron microscopy data from the 1980s of the first complete C. elegans connectome—a map of all the neurons and connections in the brain. While electron microscopes can take extremely detailed images of small samples, living organisms cannot survive the conditions inside the microscope. Most microscopes that create high resolution imagery require a lot of light, which usually damages or kills living samples, and electron microscopes require that a sample be placed in a vacuum—an environment in which very few living things can survive.
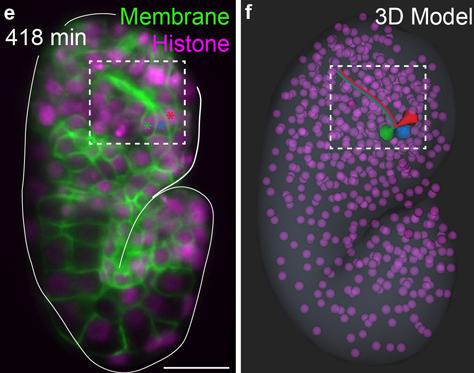
Shroff’s recent advancements, including dual-view inverted selective plane illumination microcopy (diSPIM), have allowed researchers to look at living organisms at high resolution with minimal damage to the sample. He was able to accomplish this by using a second view of the sample. In its most basic form, SPIM uses a thin sheet of light that moves relative to the sample—only exposing the area to light as it is being imaged, instead of the whole sample at once. By adding additional optics and creating computer software that combined the views from the two lenses, Shroff was able to dramatically improve the resolution of the microscope while maintaining rapid imaging speed—without significantly increasing exposure to light. This made it easier to clearly visualize the neurons in the worm embryo.
When he developed the diSPIM technology in 2013, he helped translate the technology for Colón-Ramos and his team, who eventually built an improved version in their lab. This enabled them to observe the connectome in real time. Thanks to this new diSPIM technology, the researchers could now image the orchestrated dance that coordinates the assembly of the C. elegans brain. Seeing how the neurons developed helped them to understand factors that are important in organizing brain structure and coordinating its development.
“Partnerships between tool-builders and biologists are not always easy, but often they lead both to improvements in tools and biological discovery,” Shroff said. “I’m very happy that is what happened in this case, and grateful that our decade-long collaboration is paying off.”
The microscope technology allowed the researchers to observe the position, migration, and outgrowth of neurons that make up the structure of neuropils—areas of the nervous system that are especially dense with neurons. Neuropils can be found throughout the nervous system of animals—from the brain and spine to the olfactory system. The researchers were able to track specific neurons and observe as “pioneer” neurons essentially laid down tracks during the development of the C. elegans nervous system that allowed other neurons to follow and form neuropils. For the first time, they were able to reveal some of the fundamental structural-design principles of neuropils and observe how they are organized and built by pioneer neurons.
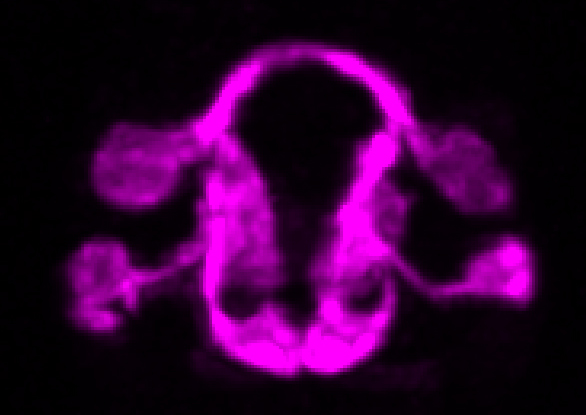
“There is a universe of rich and sophisticated biology underlying the precise wiring of the C. elegans brain,” said Colón-Ramos. "The collaborative work we have completed, and the systems and frameworks we have established, now allow us to examine the systematic assembly of a neuropil and to understand organizing principles that are necessary for coordinated circuit assembly.”
These unique observations of a living C. elegans brain being constructed would not have been possible without collaborations that stretched across fields, institutions, and decades. It has enabled researchers to watch the brain development repeatedly, which ensured that they were capturing patterns and not just a one-time occurrence. As researchers look for the answers to ever more complex questions, the resolution and speed with which microscopes are able to record data—and the resultant image processing—will need to continue advancing to keep up with the higher demand.
The work is the result of a decade long collaboration between Daniel Colón-Ramos (Yale University), Hari Shroff (NIBIB), Zhirong Bao (Sloan Kettering Institute); and William A. Mohler (University of Connecticut Health Center), all part of the consortium WormGUIDES, as well as Smita Krishnaswamy of Yale School of Medicine. It was also funded by the National Institute of General Medical Sciences, the National Cancer Institute, and the National Institute of Neurological Disorders and Stroke.