- What are tissue engineering and regenerative medicine?
- How do tissue engineering and regenerative medicine work?
- How do tissue engineering and regenerative medicine fit in with current medical practices?
- What are NIH-funded researchers developing in the areas of tissue engineering and regenerative medicine?
What are tissue engineering and regenerative medicine?
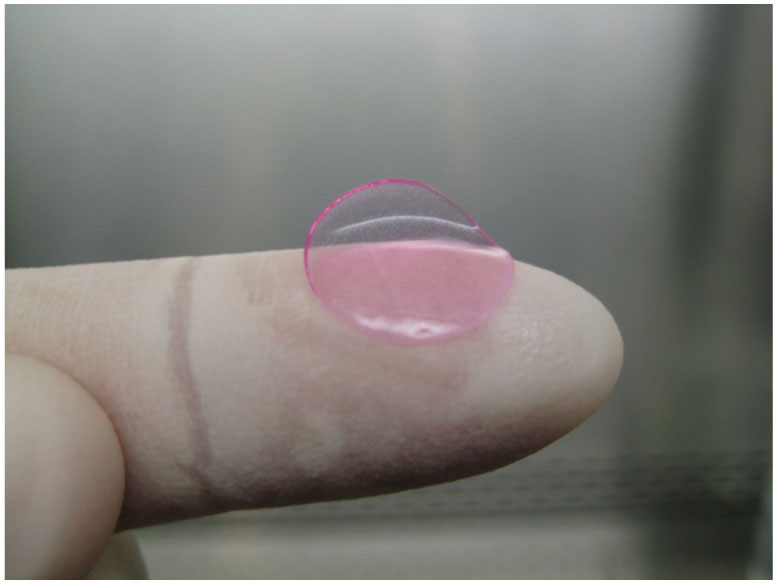
Tissue engineering evolved from the field of biomaterials development and refers to the practice of combining scaffolds, cells, and biologically active molecules into functional tissues. The goal of tissue engineering is to assemble functional constructs that restore, maintain, or improve damaged tissues or whole organs. Artificial skin and cartilage are examples of engineered tissues that have been approved by the FDA; however, currently they have limited use in human patients.
Regenerative medicine is a broad field that includes tissue engineering but also incorporates research on self-healing – where the body uses its own systems, sometimes with help foreign biological material to recreate cells and rebuild tissues and organs. The terms “tissue engineering” and “regenerative medicine” have become largely interchangeable, as the field hopes to focus on cures instead of treatments for complex, often chronic, diseases.
This field continues to evolve. In addition to medical applications, non-therapeutic applications include using tissues as biosensors to detect biological or chemical threat agents, and tissue chips that can be used to test the toxicity of an experimental medication.
How do tissue engineering and regenerative medicine work?
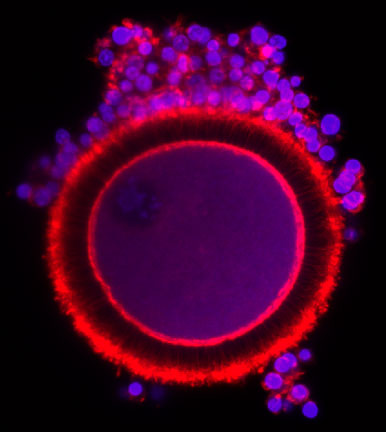
Cells are the building blocks of tissue, and tissues are the basic unit of function in the body. Generally, groups of cells make and secrete their own support structures, called extra-cellular matrix. This matrix, or scaffold, does more than just support the cells; it also acts as a relay station for various signaling molecules. Thus, cells receive messages from many sources that become available from the local environment. Each signal can start a chain of responses that determine what happens to the cell. By understanding how individual cells respond to signals, interact with their environment, and organize into tissues and organisms, researchers have been able to manipulate these processes to mend damaged tissues or even create new ones.
The process often begins with building a scaffold from a wide set of possible sources, from proteins to plastics. Once scaffolds are created, cells with or without a “cocktail” of growth factors can be introduced. If the environment is right, a tissue develops. In some cases, the cells, scaffolds, and growth factors are all mixed together at once, allowing the tissue to “self-assemble.”
Another method to create new tissue uses an existing scaffold. The cells of a donor organ are stripped and the remaining collagen scaffold is used to grow new tissue. This process has been used to bioengineer heart, liver, lung, and kidney tissue. This approach holds great promise for using scaffolding from human tissue discarded during surgery and combining it with a patient’s own cells to make customized organs that would not be rejected by the immune system.
How do tissue engineering and regenerative medicine fit in with current medical practices?
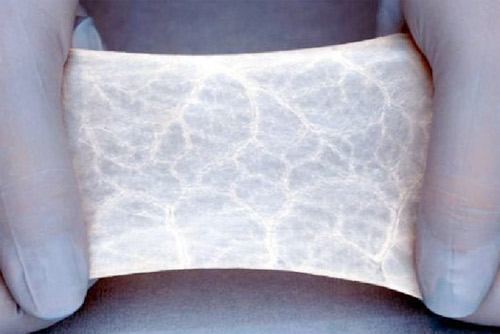
Source: Stephen Badylak, University of Pittsburgh.
Currently, tissue engineering plays a relatively small role in patient treatment. Supplemental bladders, small arteries, skin grafts, cartilage, and even a full trachea have been implanted in patients, but the procedures are still experimental and very costly. While more complex organ tissues like heart, lung, and liver tissue have been successfully recreated in the lab, they are a long way from being fully reproducible and ready to implant into a patient. These tissues, however, can be quite useful in research, especially in drug development. Using functioning human tissue to help screen medication candidates could speed up development and provide key tools for facilitating personalized medicine while saving money and reducing the number of animals used for research.
What are NIH-funded researchers developing in the areas of tissue engineering and regenerative medicine?
Research supported by NIBIB includes development of new scaffold materials and new tools to fabricate, image, monitor, and preserve engineered tissues. Some examples of research in this area are described below.
- Controlling stem cells through their environment:
For many years, scientists have searched for ways to control how stems cells develop into other cell types, in the hopes of creating new therapies. Two NIBIB researchers have grown pluripotent cells—stem cells that have the ability to turn into any kind of cell—in different types of defined spaces and found that this confinement triggered very specific gene networks that determined the ultimate fate for the cells. Most other medical research on pluripotent stem cells has focused on modifying the combination of growth solutions in which the cells are placed. The discovery that there is a biomechanical element to controlling how stem cells transform into other cell types is an important piece of the puzzle as scientists try to harness stems cells for medical uses.
- Implanting human livers in mice:
NIBIB-funded researchers have engineered human liver tissue that can be implanted in a mouse. The mouse retains its own liver as well, and therefore its normal function-but the added piece of engineered human liver can metabolize drugs in the same way humans do. This allows researchers to test susceptibility to toxicity and to demonstrate species-specific responses that typically do not show up until clinical trials. Using engineered human tissue in this way could cut down on the time and cost of producing new drugs, as well as allow for critical examinations of drug-drug interactions within a human-like system.
- Engineering mature bone stem cells:
Researchers funded by NIBIB completed the first published study that has been able to take stem cells all the way from their pluripotent state to mature bone grafts that could potentially be transplanted into a patient. Previously, investigators could only differentiate the cells to a primitive version of the tissue which was not fully functional. Additionally, the study found that when the bone was implanted in immunodeficient mice there were no abnormal growths afterwards—a problem that often occurs after implanting stem cells or bone scaffolds alone.
- Using lattices to help engineered tissue survive:
Currently, engineered tissues that are larger than 200 microns (about twice the width of a human hair) in any dimension cannot survive because they do not have vascular networks (veins or arteries). Tissues need a good “plumbing system”—a way to bring nutrients to the cells and carry away the waste—and without a blood supply or similar mechanism, the cells quickly die. Ideally, scientists would like to be able to create engineered tissue with this plumbing system already built in. One NIBIB funded researcher is working on a very simple and easily reproducible system to solve this problem: a modified ink-jet printer that lays down a lattice made of a sugar solution. This solution hardens and the engineered tissue (in a gel form) surrounds the lattice. Later, blood is added which easily dissolves the sugar lattice, leaving pre-formed channels to act as blood vessels.
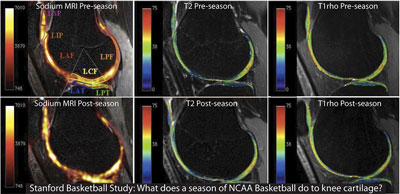
- New hope for the bum knee:
Until now, cartilage has been very difficult, if not impossible, to repair due to the fact that cartilage lacks a blood supply to promote regeneration. There has been a 50% long-term success rate using microfracture surgery in young adults suffering from sports injuries, and little to no success in patients with widespread cartilage degeneration such as osteoarthritis. An NIBIB-funded tissue engineer has developed a biological gel that can be injected into a cartilage defect following microfracture surgery to create an environment that facilitates regeneration. However, in order for this gel to stay in place within the knee, researchers also developed a new biological adhesive that is able to bond to both the gel as well as the damaged cartilage in the knee, keeping the newly regrown cartilage in place. The gel/adhesive combo was successful in regenerating cartilage tissue following surgery in a recent clinical trial of fifteen patients, all of whom reported decreased pain at six months post-surgery. In contrast, the majority of microfracture patients, after an initial decrease in pain, returned to their original pain level within six months. This researcher worked in collaboration with another NIBIB grantee to image the patients who had undergone surgery enabling scientists to combine new, non-invasive methods to see the evolving results in real-time.
- Regenerating a new kidney:
The ability to regenerate a new kidney from a patient’s own cells would provide major relief for the hundreds of thousands of patients suffering from kidney disease. Experimenting on rat, pig and human kidney cells, NIDDK supported researchers broke new ground on this front by first stripping cells from a donor organ and using the remaining collagen scaffold to help guide the growth of new tissue. To regenerate viable kidney tissue, researchers seeded the kidney scaffolds with epithelial and endothelial cells. The resulting organ tissue was able to clear metabolites, reabsorb nutrients, and produce urine both in vitro and in vivo in rats. This process was previously used to bioengineer heart, liver, and lung tissue. The creation of transplantable tissue to permanently replace kidney function is a leap forward in overcoming the problems of donor organ shortages and the morbidity associated with immunosuppression in organ transplants.